Raoul Kopelman and Rodney R. Agayan
New photodynamic cancer therapies combine the tumor detection and imaging
capabilities of nanoparticles with the increased penetration depth of infrared
two-photon excitation.
Traditional photodynamic therapy (PDT) is based on photoactive drugs that
produce excited singlet-state oxygen molecules from local (ground-state)
oxygen molecules under illumination with visible light.
1
The singlet oxygen produces reactive oxygen species, primarily free radicals
such as ·OH. These free radicals attack DNA and other vital biomolecules in
the cell, leading to cell death. Due to the limited tissue penetration of
visible light, PDT has mostly been utilized for skin cancers.
Often injected into the area of the tumor, the photodynamic drug is composed
of non-toxic molecules that efficiently absorb red wavelengths. Excitation
of these molecules, usually a triplet state excitation deriving its energy
from the excited singlet state, is quenched by oxygen molecules through an
energy exchange that excites the oxygen molecule from its ground triplet
state to its excited singlet state. This “singlet oxygen” is often called
“killer oxygen,” as it quickly reacts with neighboring molecules, including
water, to produce highly-reactive free radicals that, in turn, kill the
cell. The United States Food and Drug Administration (FDA)-approved PDT drug
is made of red blood cell hemoglobin-based molecules (trade name Photofrin),
but other PDT molecules are awaiting FDA approval, and several are approved
in Canada and Europe.
PDT has three primary problems. First, PDT is limited to superficial tumors,
or tumors that are reachable by optical fibers. Second, the PDT drug
molecule is not very selective and thus kills neighboring healthy cells.
Third, a lesser concern is the photo-toxicity of the drug; treated patients
must be kept in the dark for days or longer. At the same time, PDT has
several advantages: the therapeutic laser beam can be selectively aimed at
the tumor only; the visible radiation (usually 488nm or 633 nm) will not
penetrate far beyond the tumor, even if non-tumor cells contain the drug;
and PDT will not cause damage to cells that neither the light nor the drug
reach. Thus, compared to chemotherapy and radiation therapy, PDT is quite
benign. The question that now arises is whether we can extend this approach
to the treatment of non-superficial tumors or to tumors that are diagnosed
non-visually (with later confirmation by biopsy).
Some researchers have suggested the use of infrared active dyes in PDT,
2
as the activating infrared photons can penetrate deeper into tissue than
visible light. An analogous approach involves pulsed infrared photons used
in combination with two-photon-absorbing PDT drug molecules.
3
While this method also provides deeper photon penetration, it exacerbates
the non-selectivity of the method as well: more non-tumorous tissue will be
harmed.
The new PDT paradigm addressed here consists of nanoplatforms, which are
nanoparticles (NP) that combine PDT with tumor detection and tumor imaging
(see Figure
1). These NP, when injected into the
bloodstream, find the cancer cells and enter them using selective molecular
targeting. At the same time, they enable external MRI or optical imaging of
the tumor. This allows one to aim a laser, via an optical fiber, at the
tumor.
Figure 1. Schematic of a photodynamic nanoplatform for cancer
therapy.
The NP PDT approach has been demonstrated
4 on
rats bearing the deadliest human brain cancer, 9L Glioma. Control mice with
5mm brain tumors survive only one week; those treated with existing
therapeutic approaches live less than two weeks. But 60% of NP PDT treated
rats were cured of their brain tumors. The procedure involved only 5min
illumination by a 1W helium-neon laser, using a fiber inserted through the
skull with a hemispherical diffuser.
With the same tumor, survival time for humans is only 4–6 months, and
neither chemotherapy nor radiation therapy are effective. More details on
these nano-drugs, including their synthesis, composition, and toxicology,
are given in a paper by Yong-Eun Koo et al.
5
The latest additions to these “photonic drugs” are PDT NPs that utilize
two-photon excitation.
6 Using an experimental
two-photon PDT dye (embedded in the NP) and a pulsed Ti:sapphire laser, we
demonstrated the effectiveness of this technique on live breast-cancer cells
(see Figures
2 and
3).
Figure 2. Demonstration of cell-kill induced by two-photon
photodynamic excitation. Fluorescent cell stains indicate live cells (calcein,
green) or dead cells (propidium iodide, red). (a-d) Rat C6 glioma cells
exposed to a 100mW/cm
2, 780nm laser for 1min. Images were taken
at different times: (a) before irradiation; (b) immediately after
irradiation; (c) 40min after irradiation; (d) 120min after irradiation.
(e-h) Rat C6 glioma cells incubated with 1mg/mL two-photon
photosensitizing dye-encapsulated nanoparticles and exposed to a 100mW/cm
2,
780nm laser for 1min. Images were taken at different times: (e) before
irradiation; (f) immediately after irradiation; (g) 30min after
irradiation; (h) 130min after irradiation
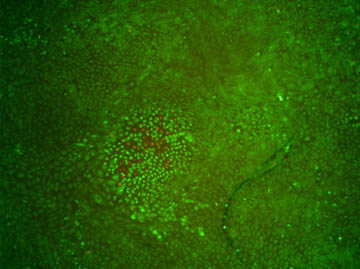
Figure 3. Demonstration of cell-kill localization. Imaging the
cells with 10×-reduced magnification after excitation reveals that only
cells in close proximity to the two-photon excitation focus are affected.
This is indicated by both red fluorescence and a change in shape of the
cells. Image acquisition occurred 135min after irradiation.
Once more-efficient two-photon dye-based NP are perfected, a second
generation of such nano-drugs should work not only for highly-transparent
brain tumors but also for other tumors in areas of the body with lower
optical transparency.
Department of Chemistry, Department of Physics, Department of Applied Physics,
Rodney R. Agayan
2. G. L. Li, A. Graham, Y. H. Chen, M. P. Dobhal, J. Morgan, G. Zheng, A.
Kozyrev, A. Oseroff, T. J. Dougherty, R. K. Pandey,
Synthesis, comparative photosensitizing efficacy, human serum albumin
(Site II) binding ability, and intracellular localization characteristics of
novel benzobacteriochlorins derived from vic-dihydroxybacteriochlorins,
J. Med. Chem. 46, no. 25, pp. 5349-5359, 2003.
doi:10.1021/jm030341y.